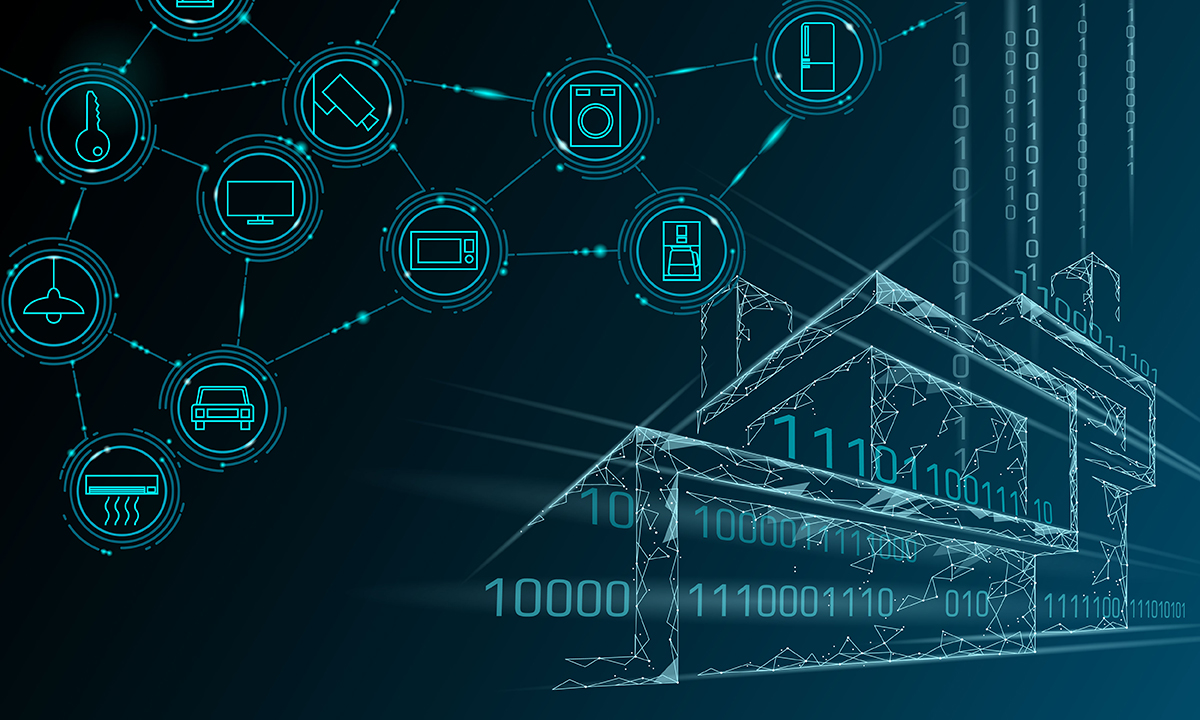
The world continues to embrace the Internet of Things (IoT), and emerging technologies are taking on a growing importance within built environments.
A confluence of innovation in big data processing, ultra-low power wireless networks, embedded sensor technology, and energy management has accelerated the emergence of smart buildings. As these become widespread, we have witnessed a reciprocal, or better yet, exponential growth in the planning activities to successfully introduce the sophisticated automation and enhanced user experiences they promise. In particular, hospitals, commercial offices, entertainment, retail, airports and education facilities all have clients who will be directly impacted by these advances in technology. This paper highlights the opportunities to provide a proactive change management plan for a redevelopment or capital project.
A redevelopment project provides an opportunity to introduce a large range of new technologies; however, the ‘big bang’ approach that is associated with the opening of a new facility can hinder the adoption. The role of technology should be understood from a functional perspective long before the walls and bricks are in place, so that proper infrastructure exists to support the smart building.
Any successful Digital Strategy and Transformation Project must consider aligning a change management approach to engage users to be prepared for opening day. The vast amounts of change can overwhelm staff when they move into a new building with new technologies, from new floor layouts and different staffing models, to the introduction of more mobile technology, more paperless systems, and automation of tasks that staff previously performed manually. It is imperative to address the capacity for change well in advance of the Opening Day.
The principles of Change Management and what is unique about a redevelopment project
Change really occurs when it is done at scale – throughout the organization - across all levels and stakeholder groups. There are several industry-recognized principles listed below for adopting change in large organizations. However, given the degree of complexity, number of stakeholders and length of project, there are unique factors that need to be considered with redevelopment.
The Principles
Change is Rolled Out
Redevelopment Project Considerations
Redevelopment projects have many external stakeholders as well as internal stakeholders; employees and project delivery teams have creative authority that can turn into resistance
Change Starts at the Top
The redevelopment project cycle covers many years and the leaders may change; executives are often insulated from the reality of day-to-day operations by layers of the organization
Change is Engineered
A change management program can be planned, coordinated and monitored; however, it is not like a construction project in that it involves breaking new ground and cannot be predetermined fully in advance
With So Many Stakeholders, What Matters Most?
With the variety of stakeholders involved in a capital project, such as end-users, executive team, information technology department, facilities/operations teams, government oversight and taxpayers, there are differing and opposing drivers for each of these groups, which include expected benefits, cost containment and scope definition. There is a need for a framework to define a course of action and for leadership to remain committed to it. Finding the common goals between all the stakeholders will be critical to the long-term success.
In a redevelopment project, the ideas and inspiration for change often come from parties outside the end-user stakeholder groups, such as the design team, the information technology department, facilities engineers, or other support services. Ideally, these ideas are then sponsored by the executive leadership with input from users; however, this is not always the case. It often happens that use cases for the functionality of technology are brainstormed by someone “higher up” or by the IT department, and then rushed straight into design. There is no wrong party to support idea generation; however, the important component is to ensure that end-users have been engaged and have faith that the new technologies will create a better environment. One method of engaging these users is to visualize the changes, and to write and approve the use cases for their workflows. This approach uses Lean thinking and iterative cycles to build consensus. It is critical that time be set aside to ensure that these use cases are considered by end users and validated.
It is important to note that not all parties will see the changes as necessary, especially if they don’t belong to the organization or share the same vision. To address this, create a cross-functional project team, map out the impacted stakeholders and address their unique needs. You will likely appreciate that some people are not able to easily adapt to new technologies. Doing so requires both willingness and capability; mindsets get in the way of actually making use of the technology. Therefore, it helps to have champions and support available. There will also be employees in the organization who are very keen to embrace change that results in a more automated and sophisticated building. Support these individuals in advance of the redevelopment projects by leading change on a smaller scale; for example, by introducing new mobile technologies or smart boards in meeting rooms.
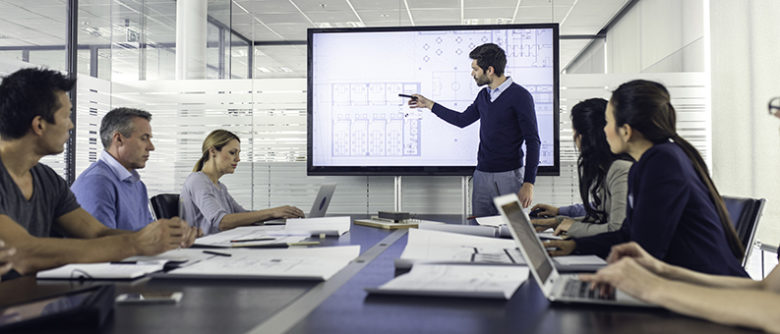
Change Starts at Every Level
IT IS IMPORTANT THAT EMPLOYEES
CONTINUE TO SEE AN IMPACT AND BE
INVOLVED IN KEEPING UP THE MOMENTUM.
Long before shovels are in the ground, the organization’s leaders are visioning what the new facility will look like and how it will operate. However, project cycles of up to 10 years can be a significant deterrent for senior leaders seeing their vision through to completion. On one hand, they may perceive what seems to be ample time to prepare for the coming changes; on the other hand, they may also feel that getting ready for a change so far in the future is futile. Therefore, it is important that employees continue to see an impact and be involved in keeping up the momentum.
Communication and setting the stage for the ultimate change may be the most critical factor in successful deployments. This requires an engineered approach to obtaining buy-in. To ensure the cultural ‘soil’ is ready before planting the seeds of change, develop a bi-directional communications plan that allows questions to be addressed. The objective is to prepare employees to understand the benefits of the change, as well as the necessity of the change, and for them to be emotionally ready to execute the change. This requires a two-way dialogue to give staff sufficient time to provide feedback. Employees who fully support the change can be invited to co-develop a plan to describe the benefits and address concerns with sufficient support and training.
Conclusion
FACILITATING CHANGE DOESN’T NEED TO
BE DIFFICULT OR ONEROUS.
As a final consideration, recognize that silos in your organization may create barriers to disseminating your plans. I have often seen change initiatives fall apart when different groups that are equally impacted refuse to take ownership for action. They wait for the other department to come up with a plan and take the lead, while their own group sits back and provides “constructive criticism”. This reveals a culture that is resistant to change. It is important as a leader to break down these barriers. Bring employee groups together to understand the shared objectives and then identify what barriers may get in the way. It may be that both departments are experiencing the strain of increased workload from a large volume of change. However, facilitating change doesn’t need to be difficult or onerous. Following Lean principles, create small batches of work, and plan to stretch these batches out over time.
6 Steps to Successful Technology Change Management
As a strong leader, you can set the stage for successful technology change by adopting these six Change Management steps:
- Identify the common goals between all stakeholder parties
- Engage end users in depicting the use cases for technology
- Communicate the benefits of these use cases
- Recognize change champions and providing them with support and training
- Test technologies in advance by using pilot studies
- Bring together stakeholders to voice their concerns
A change management program needs to be adapted to its unique situational factors. Multiple stakeholders - from financiers, end users, IT, facilities, architects and engineers - can make implementation of your plan more challenging, but by following the steps above, you can ease the process.
If you have questions or would like to learn more about change management, we are happy to start a conversation to see how we could help.
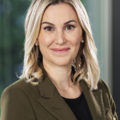
Megan Angus, RN, Lean, EDAC
Division Director, Angus Connect
megan.angus@hhangus.com