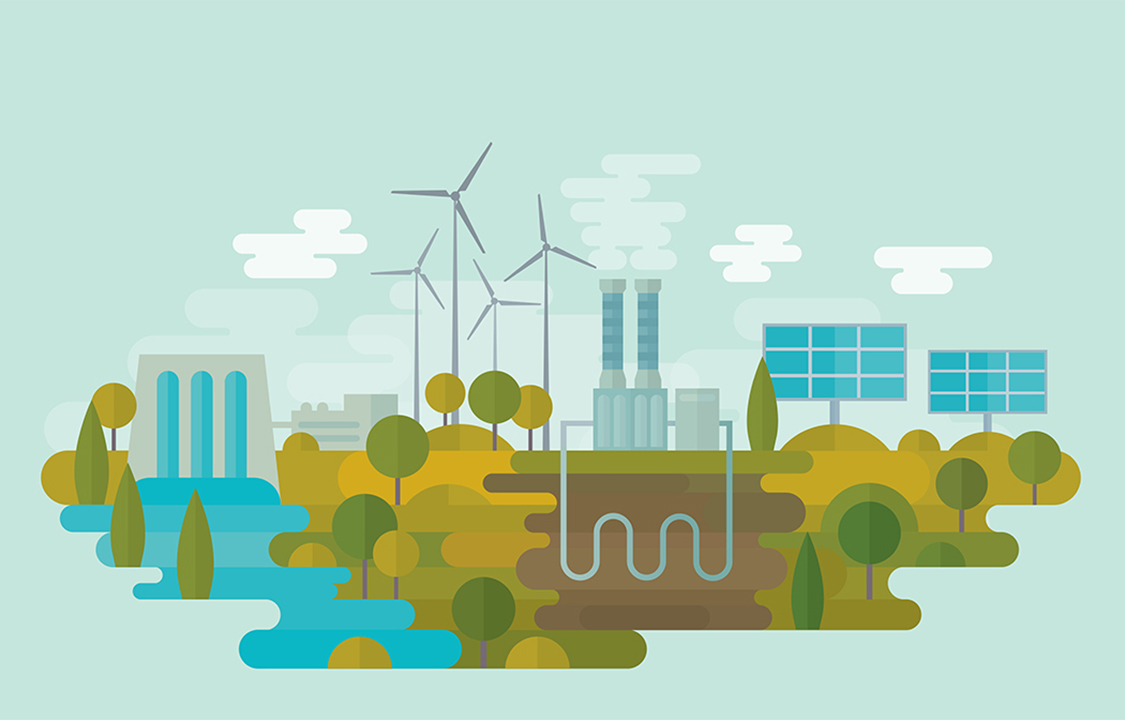
Low and zero carbon energy presents a substantial opportunity for the world. It will deliver significant benefits to the human health, well-being and prosperity, while improving the environment and sustainability of our planet.
The promise of harnessing emission-free energy is an engineering and economic opportunity that is hard to pass on. While eliminating carbon emissions has its health benefits to humans as we reduce air pollution and improve air quality in cities, the transformation to renewable and emission-free energy will help achieve a truly sustainable energy future for the world. This zero carbon energy revolution is coming. It will deliver jobs and reduce the impact of global warming on a wide variety of other important aspects of life. A Low Carbon Energy Transformation is a key component for an effective strategy to reduce greenhouse gases and boost energy security.
The Issue: Climate Crisis
Climate change took centre stage in 2019 as advocates around the world organized events and demanded government action to address the climate crisis. In 2018, the Intergovernmental Panel on Climate Change (IPCC) recommended to the United Nations that the world limit global warming to 1.5 °C (2.7 °F) above pre-industrial levels in order to avoid adverse effects on both humans and the environment. This target is possible, but would require the world reaching zero carbon emissions by the year 2050, as well as fast-tracked and extensive changes in all aspects of society.
Looking to the future, the global population will reach 10 billion by 2050, according to the World Bank. In parallel, the world’s demand for raw materials could double by 2060, according to the Organization for Economic Co-operation and Development. These factors, among others, introduce substantial pressure on the path to zero carbon energy. In order for the world to reach its climate targets, the main sources of emissions to be addressed are human activities related to transportation, agriculture, manufacturing, and buildings. For the latter, achieving near-zero/zero carbon emissions involves tackling multiple aspects, such as building construction and retrofitting activities, building envelopes, and building energy systems.
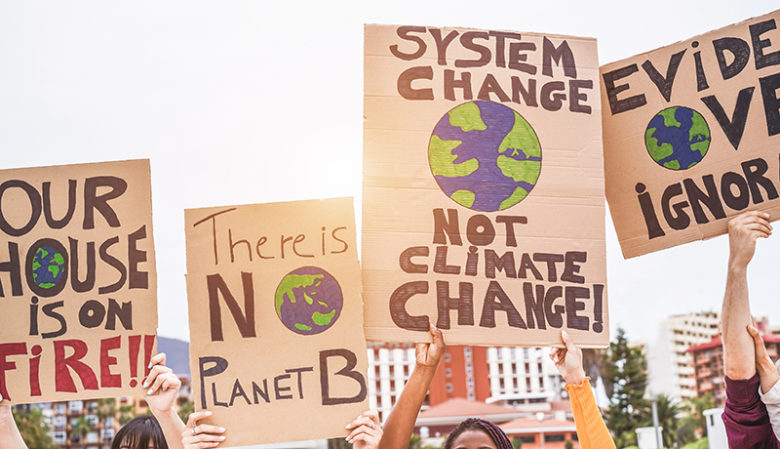
Challenging the Status Quo
Across Canada, one of the primary approaches to building heating is through fossil fuel combustion. Natural gas and fuel oils are burned to produce steam or hot water which are used for heating, or fuel is burned to heat air and sending it directly into buildings. As for cooling, buildings traditionally use refrigeration systems that rely on electricity from the grid, which may/may not use fossil fuel to generate electricity. In addition, when it comes to electric vehicles (EV), most buildings have yet to install EV charging infrastructure.
Reducing building emissions requires a focus on building energy systems, efficiencies, and strategies in order for buildings to achieve true zero carbon emissions. While ASHRAE standards 90.1 and 90.2 address building efficiencies, new smart and innovative building systems for heating and cooling must become mainstream in order to make tangible progress toward a zero carbon world.
Engineering Solutions
The good news for building emissions is that there is a wide variety of engineering solutions and strategies available to provide emission-free heating and cooling. Building owners, collaborating with engineering consultants, face the critical task of establishing evaluation criteria for each proposed emission-reduction solution or strategy, in order to determine which is most appropriate under constraints such as budget, time, and performance, and other practical considerations.
Heat Pumps
One solution is heat pump systems that can be used to satisfy both building heating and cooling loads. While heat pumps are typically powered by electricity, it is worth noting that, in Ontario, approximately 90% of electricity comes from low/zero greenhouse gas sources and has one of the lowest annual average emissions factors in Canada (31 g CO2eq/kWh electricity consumed). Heat pump systems commonly have a wide range of capacities. For heating, such systems are capable of providing heating capacities up to 30,000 MBH (8792 kW) per heat pump, and hot water of temperatures as high as 150°F (65.5°C). For large cooling loads, heat pumps have cooling capacities from 250 tons of refrigeration (TR) (879 kW) up to 1800 TR (6330 kW) per heat pump. These capabilities make heat pumps suitable for simultaneous heating and cooling of buildings in the shoulder seasons.
Heat pump systems are also capable of providing full building heating in winter when they operate in conjunction with an appropriate heat recovery system. For peak heating loads, these heat pump systems can operate side by side with low emission condensing boilers in a low carbon scenario, or coupled with thermal energy storage (TES) systems in a zero carbon scenario. It is worth noting that, for colder climates, supplemental heating may be required to satisfy peak winter loads in order to achieve zero carbon building heating. This may be achieved with other zero carbon heating alternatives. For peak cooling loads, heat pump systems work in conjunction with high efficiency centrifugal peaking chillers or thermal TES systems. Heat pumps that use carbon dioxide as a refrigerant (R744) can provide both heating and cooling emission free, and could be a promising solution if they become mainstream.
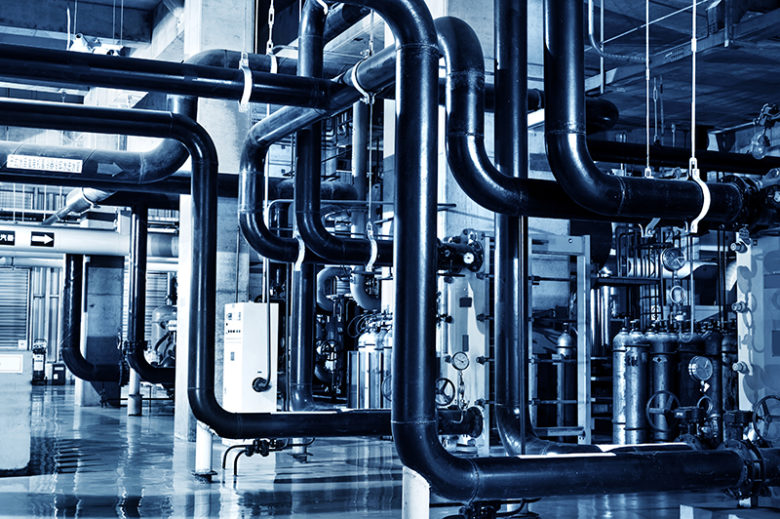
Solar Photovoltaic
Regarding on-site electricity generation, low/zero carbon electricity generation can be achieved by using solar photovoltaic (PV) systems coupled with battery storage in a zero-carbon scenario, or by utilizing small-scale low emission natural gas engines for electricity generation, which can also be coupled with battery or thermal storage in a low carbon scenario.
It is important to note that PV system capital costs have been falling dramatically in the past few years, with solar panel efficiencies up to 23%. In addition, battery storage system prices are becoming more competitive. They continue to decrease in cost and are destined to play a significant role in this market.These economic factors will significantly contribute to the zero carbon transformation for reducing building on-site emissions, as they will help to make projects financially viable.
For large-scale integrated applications, such as neighborhood-scale heating/cooling systems or institutional campuses, buildings can utilize an ambient water loop that operates between 50°F and 75°F (10°C and 20°C), or a resilient redundant thermal grid. This system uses an underground pipe network to supply a heat source/sink capability, which is then coupled with individual heat pumps in each building to either draw heat from the loop or inject heat into the loop. One caveat is that this may require retrofits for some buildings in order to accommodate lower water temperatures for heating. These retrofits may involve implementing measures such as re-insulation of the building envelope, high performance glazing, and upgrading the heating and cooling systems in the buildings.
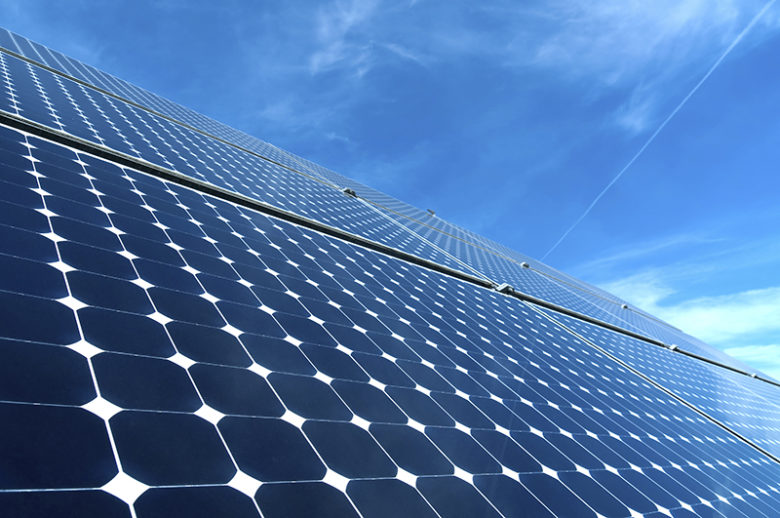
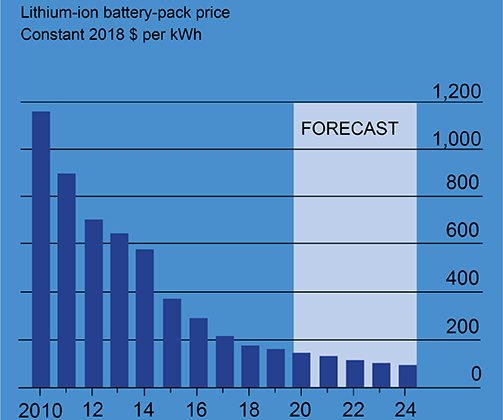
Energy Storage
On-site energy storage systems, such as battery storage or TES, assist both electrical and thermal grids in satisfying peak demand and increasing overall system reliability. Heat recovery is a similar solution that helps achieve zero carbon. These systems work by reclaiming/dispensing thermal energy from/to sources like wastewater, storm water, and open bodies of water.
Geo-exchange
For building heating and cooling, geo-exchange thermal energy is supplied to or extracted from the earth’s surface. The advantage of geo-exchange is that the earth’s temperature is stable over time; for example, in some regions of the world, soil temperature below the frost line remains a constant 45°F-50°F (7°C - 10°C) year-round. In other words, geo-exchange uses Earth’s outer layers as a rechargeable thermal battery. This strategy works best in specific climates and involves geotechnical, civil works, and landscaping considerations.
Geothermal Energy
Another promising solution is Geothermal Energy (which differs from geo-exchange). It uses thermal energy from deeper layers of the earth (2500 meters+) to provide higher temperature heat that could be used for process heat or to distribute thermal energy for heating on a larger scale.
Small Modular Reactors (SMR)
SMRs can be safely deployed in remote areas and would provide carbon-free electricity up to 600-1200 MWe per unit, in parallel with high-grade process heat (up to 1112°F (600°C) for capacities up 1.5 Billion BTU), or heat that can be used for city-wide heating.
The Challenges Ahead
Implementing any of the above-mentioned solutions carries challenges, the main one being economics. Any zero carbon solution has to deliver a competitive return on investment for cost per unit of energy, total capital cost, operational cost, and marginal cost for system reliability for mission-critical applications. These emission-free solutions may, however, offer future economic advantages when compared to traditional methods.
The advantages become clear when considering economic risk factors such as carbon pricing, cost of depreciation of assets due to regulation, and legislative risk, as well as cost savings of new zero carbon technologies arising from future technological disruption. For example, the heat pump market has been changing rapidly in the past two years (2018-2020), introducing large-scale heat pump systems at lower cost which makes them financially competitive.
Other challenges to zero carbon energy solutions may prove more problematic; for example, the challenges of business repositioning for some energy stakeholders, such as fossil fuel energy producers, distributors, resellers, and equipment vendors. Repositioning businesses to benefit from the zero carbon transformation can induce substantial resistance to change, perhaps due to accelerated time frames, as well as human capital problems, or due to changing demands for skills in the job market.
Planning and deploying an effective energy strategy, including creating and implementing resilient and adaptive energy roadmaps that can actively respond to changing economic and environmental conditions, is a solid start to a zero carbon energy transition.
Our highly skilled energy consultants are available to discuss low/zero carbon energy options and the transformation solutions best suited to your needs.
For more information, contact lowcarbon@hhangus.com
Resources:
Summary for Policymakers – IPCC
https://www.ipcc.ch/2018/10/08/summary-for-policymakers-of-ipcc-special-report-on-global-warming-of-1-5c-approved-by-governments/
A Clearer View on Ontario’s Emissions - The Atmospheric Fund,
https://taf.ca/publications/a-clearer-view-on-ontarios-emissions-2019/
Deep Lake Water Cooling System - ACCIONA https://www.acciona.us/projects/construction/port-and-hydraulic-works/deep-lake-water-cooling-system/
Geothermal energy - IRENA https://www.irena.org/geothermal
Author:
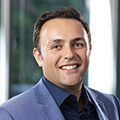
Mike Hassaballa, MASc., P.Eng.
lowcarbon@hhangus.com